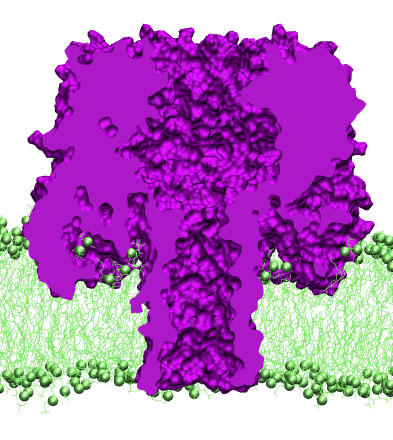
In a biological cell, membrane channels act like miniature valves regulating the flow of ions and other solutes between intracellular compartments and across the cell's boundary. Assembled in complex circuits, they generate, transmit, and amplify signals orchestrating cell function. To investigate how membrane channels work, a tiny patch of a cell membrane is isolated using an extremely fine pipette, and, in so-called patch clamp measurements, electric currents in response to applied electric potentials are determined. Dramatic increase in computational power and its efficient utilization by a massively parallel molecular dynamics code allows one today to reproduce such studies computationally, calculating the permeability of a membrane channel to ions, water, and solutes directly from its atomic structure. In what was one of the largest molecular dynamics simulations in 2005, one copy of the membrane channel α-hemolysin, submerged in a lipid membrane and water, was subject to an external electric field that drove ions, water, and DNA through the channel. Repeating simulations at several voltage biases yielded the current-voltage curve of α-hemolysin and a set of electrostatic potential maps. We observed sensitivity of the channel conductance to the solution pH, computed osmotic permeability of water through the transmembrane pore, and estimated water and ion fluxes through the side channels. The results of the simulations were found in excellent agreement with available experimental data. This study demonstrated for the first time the ability of molecular dynamics simulations to predict ionic conductance of a membrane channel starting from its X-ray structure. This study appeared in Biophysical Journal and has been cited more than 200 times.