Percolation transition prescribes protein size-specific barrier to passive transport through the nuclear pore complex.
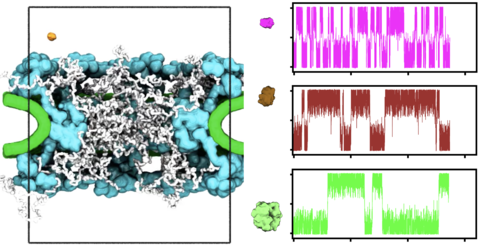
Nuclear pore complexes (NPCs) control biomolecular transport in and out of the nucleus. Disordered nucleoporins in the complex's pore form a permeation barrier, preventing unassisted transport of large biomolecules. Here, we combine coarse-grained simulations of experimentally derived NPC structures with a theoretical model to determine the microscopic mechanism of passive transport. Brute-force simulations of protein transport reveal telegraph-like behavior, where prolonged diffusion on one side of the NPC is interrupted by rapid crossings to the other. We rationalize this behavior using a theoretical model that reproduces the energetics and kinetics of permeation solely from statistics of transient voids within the disordered mesh. As the protein size increases, the mesh transforms from a soft to a hard barrier, enabling orders-of-magnitude reduction in permeation rate for proteins beyond the percolation size threshold. Our model enables exploration of alternative NPC architectures and sets the stage for uncovering molecular mechanisms of facilitated nuclear transport.
Movie 1. Equilibration simulation of the nuclear pore complex. The animation illustrates the last 1,000 microseconds of a 7,500-microsecond coarse-grained simulation. Colors identify the NPC scaffold (cyan), envelope (green), and tethered FG-nups (many colors). FGnups are colored by species.
Movie 2. Coarse grained simulation of passive diffusion. The animation illustrates a 750-microsecond fragment of a coarse-grained simulation where α-lactalbumin attempts to and eventually crosses through the NPC. The crossing even happens toward the end of the animation. Colors identify the NPC scaffold (cyan), envelope (green), tethered FG-nups (white) and αlactalbumin (orange). The cylindrical confinement potential, radius of 50 nm and height of 120 nm, is outlined in black. Lower-left black scale bar, 10 nm.
Movie 3. Illustration of a typical crossing event. The animation illustrates a 6-microsecond fragment of a much longer MD trajectory where a maltose-binding protein is seen to pass through the NPC. Colors identify the NPC scaffold (cyan), envelope (green), tethered FG-nups (white) and maltosebinding protein (red). The instantaneous coordinates of the FG-nups and of the protein were averaged over two consecutive frames (20 ns) to smooth the representation of their motion. The cylindrical confinement potential, radius of 25 nm and height of 120 nm, is outlined in black. Lower-left black scale bar, 10 nm.