Step-defect guided delivery of DNA to a graphene nanopore
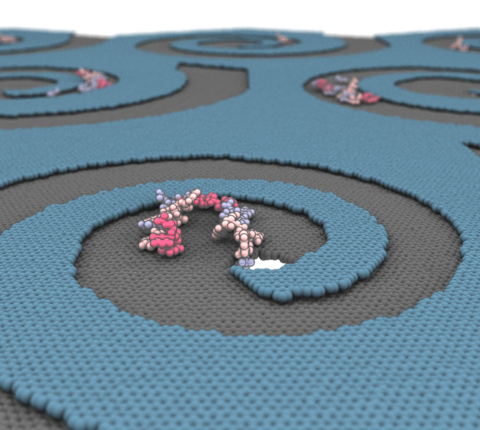
Precision placement and transport of biomolecules are critical to many single-molecule manipulation and detection methods. One such method is nanopore sequencing, in which the delivery of biomolecules towards a nanopore controls the method's throughput. Using all-atom molecular dynamics, here we show that the precision transport of biomolecules can be realized by utilizing ubiquitous features of graphene surface-step defects that separate multilayer domains. Subject to an external force, we found that adsorbed DNA moved much faster down a step defect than up, and even faster along the defect edge, regardless of whether the motion was produced by a mechanical force or a solvent flow. We utilized this direction dependency to demonstrate a mechanical analogue of an electric diode and a system for delivering DNA molecules to a nanopore. The defect-guided delivery principle can be used for the separation, concentration and storage of scarce biomolecular species, on-demand chemical reactions and nanopore sensing.
Chronological AFM images of the displacement of M13 ssDNA on freshly cleaved HOPG in air.
Chronological AFM images of the displacement of M13 ssDNA on freshly cleaved HOPG in solution.
The forced migration of poly(dT)20 down (left), up (center) and along (right), a step defect on a graphene membrane (grey). The CoM of the ssDNA molecule is attached to a spring and pulled with a constant velocity of 1.0 nm ns−1.
Directional displacement of a poly(dT)20 strand along a graphene membrane (grey) driven by water flow that periodically reverses direction. Multiple images of the unit cell are shown for clarity. The flow was produced by the application of a ±9.2 bar/nm pressure gradient. The direction of the flow alternates between pointing down (orange arrows) and up (cyan arrows) the step defect.
Forced migration of poly(dT)20 along a nanopore array in a four-layer graphene membrane (grey). Each nanopore is surrounded by three concentric single-atom step defects. The direction of the 400 pN magnitude force reverses every 10 ns. A 500 mV transmembrane bias is applied throughout the simulation.
Guided transport of poly(dT)20 to and from a nanopore at the centre of the spiral structure. The graphene nanostructure consists of a three-layer graphene membrane that has an atom-depth spiral pattern cutout in the outer two layers (yellow, blue). An external force of 300 pN magnitude is applied to the DNA molecule. The direction of the force changes by 90 degrees clockwise every 5 ns for a total of 26 constant force fragments. A transmembrane potential of 500 mV is applied throughout the simulation.
Guided transport of a 20-residue fragment of the α-haemolysin protein (residues 110 to 130) to the nanopore at the centre of the spiral structure. The graphene nanostructure consists of a three-layer graphene membrane that has an atom-depth spiral pattern cutout in the outer two layers (yellow, blue). An external force of 300 pN magnitude is applied to the protein fragment. The direction of the force changes by 90 degrees clockwise every 7.5 ns.
Guided transport of poly(dT)20 to a nanopore at the centre of a right-angled spiral structure. The graphene nanostructure consists of a two-layer graphene membrane that has an atom-depth right-angled spiral pattern cut in the top layer (blue). An external force of 300 pN magnitude is applied to the DNA molecule. The direction of the force (indicated by the arrow in the animation) changes by 90 degrees clockwise every 5 ns.
Guided transport of poly(dT)20 to a nanopore at the centre of a right-angled spiral structure of increasing depth. The graphene nanostructure consists of eight graphene layers. Each layer contains a segment of a rectangular spiral, starting from the outermost segment. The spiral pattern increases in depth by a single atomic layer with each right-angle turn. An external force of 300 pN magnitude is applied to the DNA molecule. The direction of the force (indicated by the arrow in the animation) changes by 90 degrees clockwise every 7 ns.