Water Mediates Recognition of DNA Sequence via Ionic Current Blockade in a Biological Nanopore
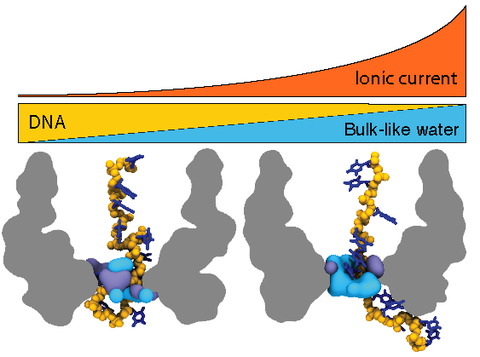
Electric field-driven translocation of DNA strands through biological nanopores has been shown to produce blockades of the nanopore ionic current that depend on the nucleotide composition of the strands. Coupling a biological nanopore MspA to a DNA processing enzyme has made DNA sequencing via measurement of ionic current blockades possible. Nevertheless, the physical mechanism enabling the DNA sequence readout has remained undetermined. Here, we report the results of all-atom molecular dynamics simulations that elucidated the physical mechanism of ionic current blockades in the biological nanopore MspA. We find that the amount of water displaced from the nanopore by the DNA strand determines the nanopore ionic current, whereas the steric and base-stacking properties of the DNA nucleotides determine the amount of water displaced. Unexpectedly, we find the effective force on DNA in MspA to undergo large fluctuations, which may produce insertion errors in the DNA sequence readout.
Molecular dynamics simulation of the full-length MspA-DNA-phi29 polymerase system under a 180 mV bias. The MspA nanopore is shown as a green molecular surface cut-away to reveal a template DNA strand threaded with its 5'-end through the MspA constriction and its 3'-end through the phi29 polymerase (semitransparent blue molecular surface). A DNA strand complementary to the template strand forms a DNA duplex between the active site of the DNA polymerase and the 3'-end of the template strand. The MspA nanopore is embedded in a POPC bilayer (purple). Water and ions are not shown. During the course of this 130 ns simulation, the polymerase and the duplex part of the DNA rotate about the axis of the pore by more than 90 degrees. Such large scale motion of the polymerase did not have a measurable effect on the conformation of the template strand in the MspA constriction.
Molecular dynamics simulation of the 5'-trans poly(dT) system. The reduced-length MspA nanopore is shown as a cut-away green molecular surface, the backbone of the DNA fragment is shown as yellow spheres, and the DNA bases are shown in blue; the lipid bilayer is shown as purple lines and spheres. Water and ions are not shown. The uppermost nucleotide of the DNA strand is harmonically restrained, which mimics the action of a DNA polymerase. This animation illustrates a 9.77 microsecond fragment of the all-atom trajectory obtained under a 180 mV transmembrane bias.
Molecular dynamics simulation of the 5'-trans poly(dC) system. The reduced-length MspA nanopore is shown as a cut-away green molecular surface, the backbone of the DNA fragment is shown as yellow spheres, and the DNA bases are shown in purple; the lipid bilayer is shown as purple lines and spheres. Water and ions are not shown. The uppermost nucleotide of the DNA strand is harmonically restrained, which mimics the action of a DNA polymerase. This animation illustrates a 8.77 microsecond fragment of the all-atom trajectory obtained under a 180 mV transmembrane bias.
Molecular dynamics simulation of the 5'-trans poly(dA) system. The reduced-length MspA nanopore is shown as a cut-away green molecular surface, the backbone of the DNA fragment is shown as yellow spheres, and the DNA bases are shown in red; the lipid bilayer is shown as purple lines and spheres. Water and ions are not shown. The uppermost nucleotide of the DNA strand is harmonically restrained, which mimics the action of a DNA polymerase. This animation illustrates a 8.46 microsecond fragment of the all-atom trajectory obtained under a 180 mV transmembrane bias.
Molecular dynamics simulation of the 3'-trans poly(dT) system. The reduced-length MspA nanopore is shown as a cut-away green molecular surface, the backbone of the DNA fragment is shown as yellow spheres, and the DNA bases are shown in blue; the lipid bilayer is shown as purple lines and spheres. Water and ions are not shown. The uppermost nucleotide of the DNA strand is harmonically restrained, which mimics the action of a DNA polymerase. This animation illustrates a 6.41 microsecond fragment of the all-atom trajectory obtained under a 180 mV transmembrane bias.
Molecular dynamics simulation of the 3'-trans poly(dC) system. The reduced-length MspA nanopore is shown as a cut-away green molecular surface, the backbone of the DNA fragment is shown as yellow spheres, and the DNA bases are shown in purple; the lipid bilayer is shown as purple lines and spheres. Water and ions are not shown. The uppermost nucleotide of the DNA strand is harmonically restrained, which mimics the action of a DNA polymerase. This animation illustrates a 5.76 microseconds fragment of the all-atom trajectory obtained under a 180 mV transmembrane bias
Molecular dynamics simulation of the 3'-trans poly(dA) system. The reduced-length MspA nanopore is shown as a cut-away green molecular surface, the backbone of the DNA fragment is shown as yellow spheres, and the DNA bases are shown in red; the lipid bilayer is shown as purple lines and spheres. Water and ions are not shown. The uppermost nucleotide of the DNA strand is harmonically restrained, which mimics the action of a DNA polymerase. This animation illustrates a 6.41-microsecond all-atom trajectory obtained under a 180 mV transmembrane bias.
Microscopic mechanism of ion passage through the MspA constriction. This animation illustrates a short (3 ns) fragment of the 5'-trans poly(dT) trajectory. The instantaneous coordinates of the system were recorded every 5 ps for this fragment of the trajectory. The reduced-length MspA channel is shown as a cut-away green molecular surface, the DNA fragment is shown in blue, the lipid bilayer in purple, and potassium and chloride ions as yellow and cyan spheres, respectively. Structural and bulk-like water molecules are shown as semitransparent blue and light grey spheres, respectively. Structural water molecules are defined as those located within 2.5 A of any protein or DNA atom. Flickering of the water molecules at the periphery of the simulation system is produced by water molecules moving in and out of the volume visualized in this animation.